+++ NEWS Alert: ERC project NANOPlot started in September 2024 +++
Who we are
We are an interdisciplinary group of scanning force microscopy (SFM) enthusiasts, constantly exploring the limits of this fascinating microscopy method. The goal is to understand the underlying physics of nanoscale systems.
One focus is on novel photovoltaic materials and devices (so-called perovskite solar cells). We develop new methods based on Kelvin probe force microscopy to map the potential and photovoltage distribution, both on photovoltaic thin films and across the different layers of operating solar cell devices. Furthermore, we were the first to report the existence of ferro-elastic domains in perovskite films.
To explore the limits of SFM, we developed a low-noise microscope. This setup is optimized for operation in liquid media and can map the surface topography with atomic resolution. Furthermore, it provides a very flexible platform for the fast implementation of new operation modes including electrical or photothermal excitation or multifrequency excitation and detection. Using this microscope we want to explore fundamental mechanisms of molecular interactions at solid-liquid interfaces.
In addition to the SFM activities, we investigate a peculiar effect called slide electrification: A water drop sliding over an insulating, hydrophobic surface will charge up electrically. Similar to triboelectricity, the voltages can be very high: up to several kilovolts. To understand this effect and to optimize the conversion efficiency, we develop sensitive measurement tools that enable reliable measurements of the small drop charge.
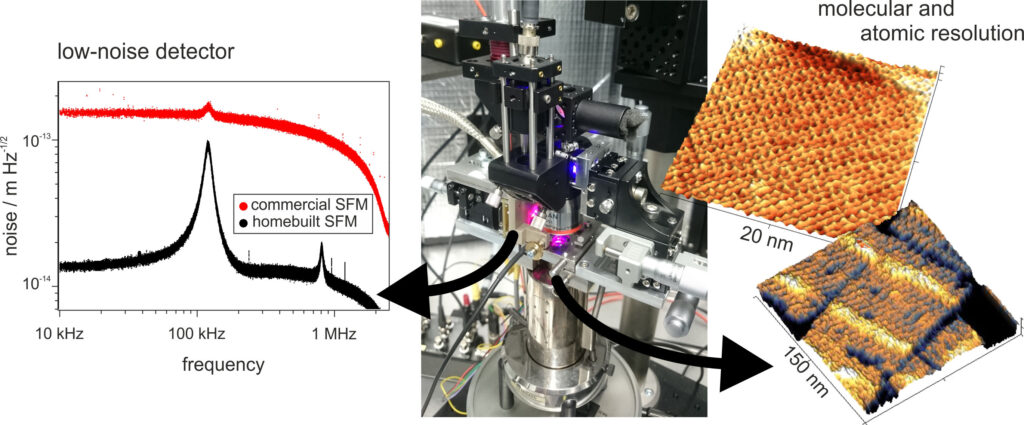
What is force microscopy?
…if until now you did not understand a single word
A scanning force microscope, often also called atomic force microscope (AFM) is a device that detects tiny forces between a tiny and very sharp tip and a sample surface. These forces originate from the interaction of the atoms in the tip apex and the atoms on the sample surface. By raster-scanning the surface, the SFM can „feel“ the structure underneath the tip and the surface topography can be reconstructed.
To detect these forces, the tip is positioned at the end of a rectangular silicon beam called cantilever that is much smaller that a human hair (see image below). Any force on the tip deflects the beam up or down. This deflection is detected by a laser beam that is reflected from the backside of the silicon beam. The limit for the noise detection is the noise in the deflection signal. This noise has two contributions:
- Detector noise: Noise caused by fluctuations in the laser intensity and the electronic noise in the detector. This contribution can be significantly reduced by the SFM design as demonstrated in the noise graph above.
- Thermal noise: thermal fluctuations let the cantilever vibrate (the two broad peaks in the noise graph above). This noise sets the fundamental detection limit for a given cantilever.
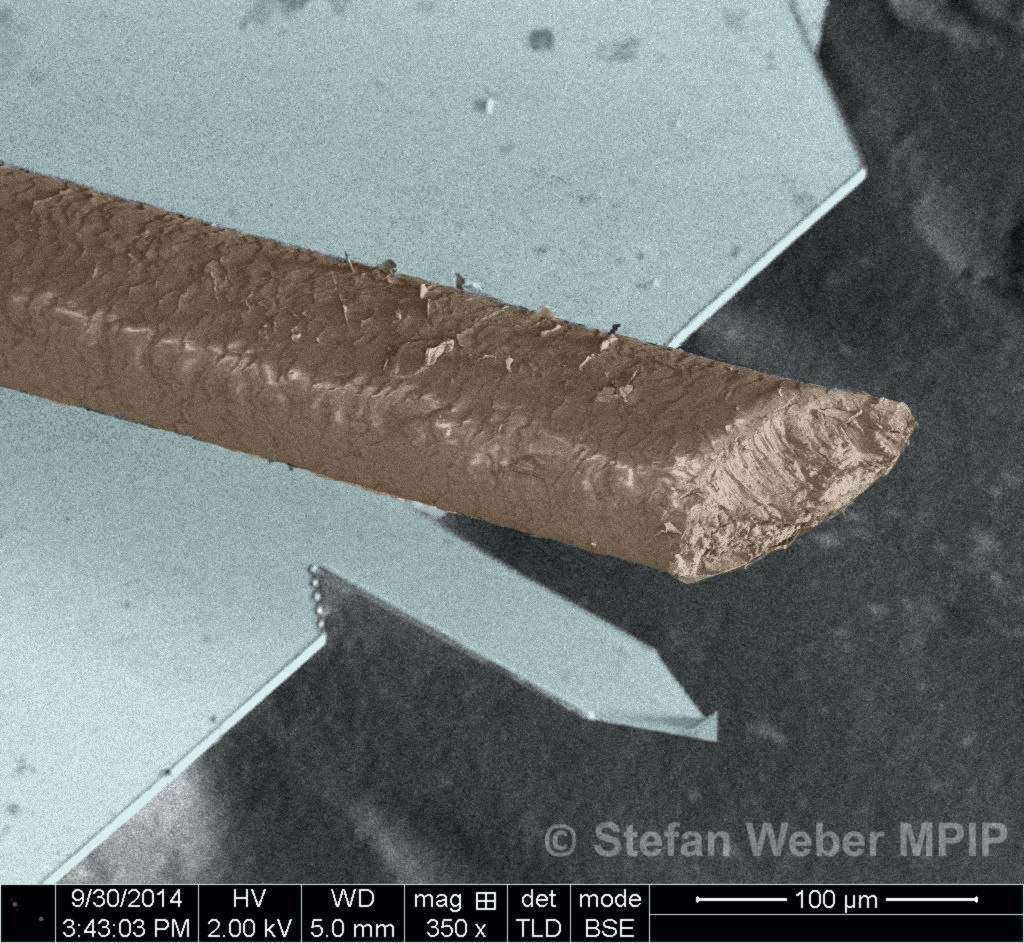